Power amplifier
Output section is a well known audio power amplifier with a complementary transistor
pair at the output. This configuration is easy to set up and adjust. It is very stable
in terms of ambient/components temperature changes and, what is the most important in
this specific application, offers low signal distortion and is able to amplify signals
down to suboudible frequencies. The subcircuit diagram is given below.
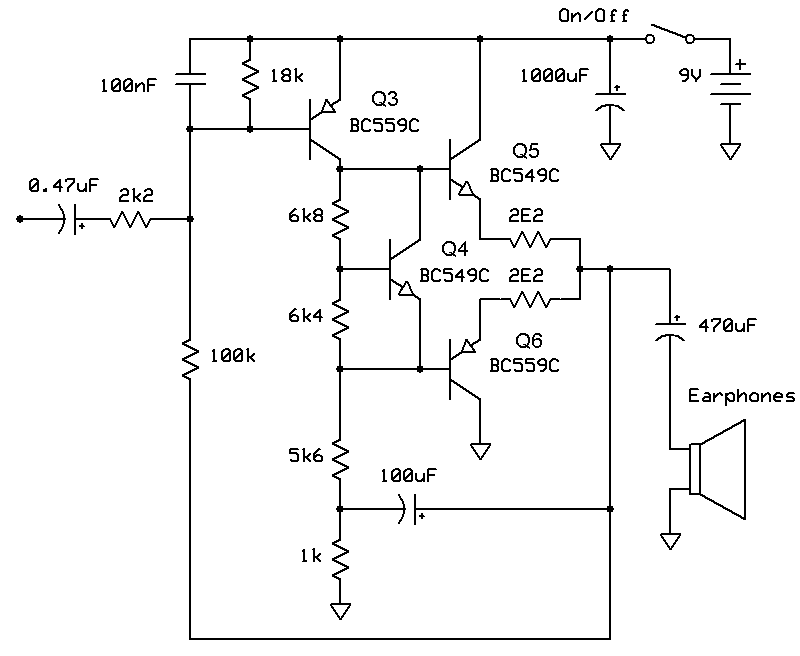
The easiest way to analyse this subcircuit is to start exploring it from its end -
the complementary output pair formed by Q5 and Q6. We can observe that each of
these two transistors works on its own as an emmiter follower i.e. an amplifier
with the common collector. Note that NPN collector is grounded to +Vcc while PNP
collector is grounded to GND. As is well known, a transistor in common collector
configuration simply translates input signal voltage from its base to its emitter,
shifted by 0.6V DC. No voltage amplification takes place, but only current amplification
equal to transistor forward current gain, usually in the order of hundreds of times.
This means that input impedance of any such transistor is very high making it easy
to be driven by a low power preamplifier, while at the same time its output impedance
is very low, sufficient for driving low impedance loads such as earphones and
loudspeakers.
In order to enable two complementary common collector transistors to work in a pair,
it is necessary to match DC output voltages at their emitter terminals. Thus we need
to provide 0.6V + 0.6V = 1.2V DC voltage difference between their bases, which is the
exact and only purpose of Q4. What it does is generating a constant voltage difference
of approximately 1.2V between its emitter and collector. The same could be achieved
using a pair of silicon diodes such as 1N4148 but this configuration enables fine
tuning of voltage in interest by adjusting the ratio between 6k8 and 6k4 resistors
around Q4: Vdiff = 0.6V x (1 + (6k8 / 6k4)). Why is this important? Because Q5 and Q6
quiescent currents depend on this voltage. By adjusting the resistor ratio we can
easily set Q5/Q6 quiescent current so that it is either not too low which leads to
acceptably low nonlinear signal distortions, but also that it is not too high which
means that there is no excess energy dissipation in the output transistor pair and
there is no unnecessary battery drain. Resistor values shown set the quiescent current
to approximately 0.5mA which is optimal for reliable power amplifier operation. Two
2,2Ω resistors inserted between output pair emitters make quiescent current very
stabile in terms of temperature and battery voltage fluctuations.
Moving further leftwards in the circuit, we observe Q3 operating in common emmiter
configuration. Q4 and its surrounding resistors serve as Q3 collector load. Q3 therefore
works as a local low power preamplifier and is also responsible for regulating
output DC voltage to optimal Vcc/2. This is done by 100k and 18k resistors which form
a voltage divider leading partial output voltage into Q3 base. It is not hard to
conclude that: Vout DC = 0.6V x (1 + (100k / 18k)). Since Q3 is PNP device, this voltage
should be calculated with respect to +Vcc potential. This resistor divider also leads
the some proportion of AC output signal into Q3 base forming a strong feedback loop
which nicely linearises residual output pair nonlinear distortions.
The final touch to the circuit design is bootstrapping Q3 collector load resistors by
the addition of 100uF cappacitor. Here it is not of interest to uppscale Q3 gain as it
is important to provide uninterrupted current flow through 5k6 resistor over the whole
AC signal cycle. As has been explained on the introductory page, lowest frequency
stethoscope signals can have rather large amplitudes; this could easily lead to observable
clipping in output signal negative semiperiods hadn't bootstrap drive been provided.
Very large expected amplitudes of output signals were also the main reason that otherwise
completely unnecessarily high power supply voltage of 9V has been chosen for this specific circuit.
Possible improvements
The whole circuit as presented here has been designed and breadboard tested in a single
weekend. Tests mainly consisted of listening to circuit output by ear, which is in essence
all that any relatively experienced hobby designer needs when playing around with audio
circuits. Everything seems to work just fine for now, but obviously there is room for
further improvements.
For example, feedback loops that include cappacitors have not been thoroughly examined.
As has been explained, circuit topology provides nice self regulation and stable operation,
but some additional fine tuning would probably make it a bit better still. The circuit
has pretty large dynamic range so signal clipping is a relatively rare event; yet due
to unavoidable mechanical shocks to the sensing surface during stethoscope usage
i.e. scratching it against skin surface, they do happen. So adding some kind of signal
compressor or AGC circuit into the signal path (between the preamplifier and output
amplifier) might not be a bad idea. I am not aware if those would cause troubles to
doctors when diagnosing patient problems so they were not included in this first test
version of the device.
Current consumption is merely 2mA which is quite acceptable for a standard alcaline 9V
battery as it is able to power the circuit for hundreds of hours continually. If we
remember that a single patient examiantion usually lasts a few minutes, this easily
translates to months if not years of regular usage. Low power consumption is the reason
that no "Power On" LED has been included - it would at least double the drain.
The least thoroughly tested circuit detail is the microphone type and the method to
attach it mechanically to acoutical parts of the stethoscope. For testing purposes,
a standard electret condenser microphone capsule has been chosen since there were a
few sitting around at the time and they seem to fit nicely into the cut rubber tubbing.
There is a possibility that dynamic microphones would work even better taking into account
the characteristic frequency spectrum of stethoscope signals - due to the physical
principle of operation, dynamic microphones have a tendency to emphasise low frequency
signals. The picture of the test device is shown on the next page together with some
recordings made with it.